Clouds scudded over the small volcanic island of Principe, off the western coast of Africa, on the afternoon of May 29, 1919. Arthur Eddington, director of the Cambridge Observatory in the U.K., waited for the Sun to emerge. The remains of a morning thunderstorm could ruin everything.
The island was about to experience the rare and overwhelming sight of a total solar eclipse. For six minutes, the longest eclipse since 1416, the Moon would completely block the face of the Sun, pulling a curtain of darkness over a thin stripe of Earth. Eddington traveled into the eclipse path to try and prove one of the most consequential ideas of his age: Albert Einstein’s new theory of general relativity.
Eddington, a physicist, was one of the few people at the time who understood the theory, which Einstein proposed in 1915. But many other scientists were stymied by the bizarre idea that gravity is not a mutual attraction, but a warping of spacetime. Light itself would be subject to this warping, too. So an eclipse would be the best way to prove whether the theory was true, because with the Sun’s light blocked by the Moon, astronomers would be able to see whether the Sun’s gravity bent the light of distant stars behind it.
Two teams of astronomers boarded ships steaming from Liverpool, England, in March 1919 to watch the eclipse and take the measure of the stars. Eddington and his team went to Principe, and another team led by Frank Dyson of the Greenwich Observatory went to Sobral, Brazil.
Totality, the complete obscuration of the Sun, would be at 2:13 local time in Principe. Moments before the Moon slid in front of the Sun, the clouds finally began breaking up. For a moment, it was totally clear. Eddington and his group hastily captured images of a star cluster found near the Sun that day, called the Hyades, found in the constellation of Taurus. The astronomers were using the best astronomical technology of the time, photographic plates, which are large exposures taken on glass instead of film. Stars appeared on seven of the plates, and solar “prominences,” filaments of gas streaming from the Sun, appeared on others.
Eddington wanted to stay in Principe to measure the Hyades when there was no eclipse, but a ship workers’ strike made him leave early. Later, Eddington and Dyson both compared the glass plates taken during the eclipse to other glass plates captured of the Hyades in a different part of the sky, when there was no eclipse. On the images from Eddington’s and Dyson’s expeditions, the stars were not aligned. The 40-year-old Einstein was right.
“Lights All Askew In the Heavens,” the New York Times proclaimed when the scientific papers were published. The eclipse was the key to the discovery—as so many solar eclipses before and since have illuminated new findings about our universe.
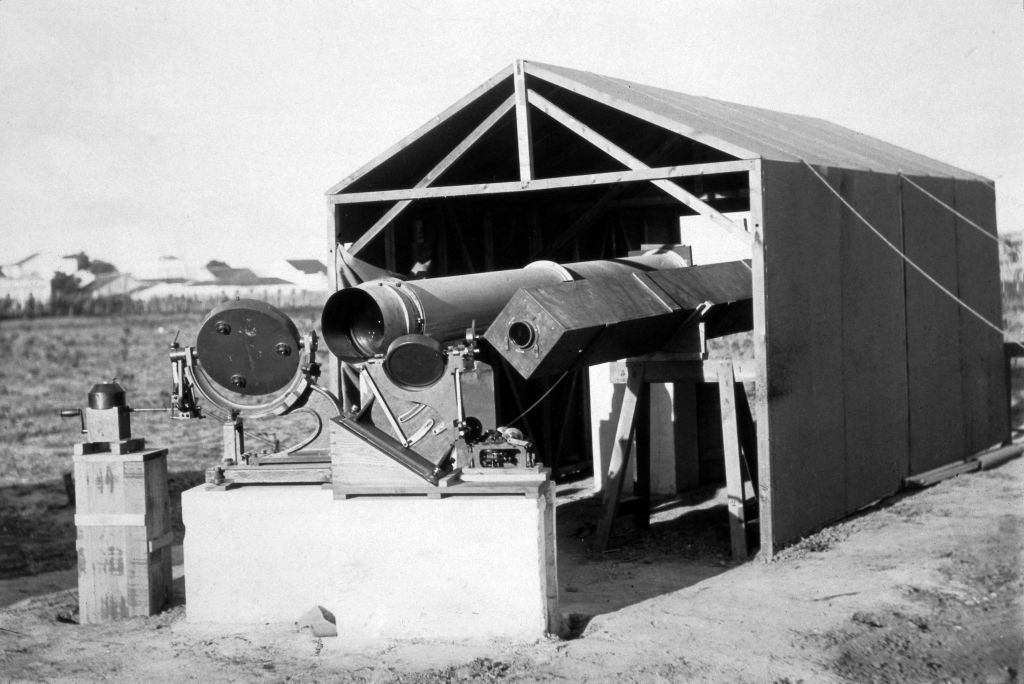
To understand why Eddington and Dyson traveled such distances to watch the eclipse, we need to talk about gravity.
Since at least the days of Isaac Newton, who wrote in 1687, scientists thought gravity was a simple force of mutual attraction. Newton proposed that every object in the universe attracts every other object in the universe, and that the strength of this attraction is related to the size of the objects and the distances among them. This is mostly true, actually, but it’s a little more nuanced than that.
On much larger scales, like among black holes or galaxy clusters, Newtonian gravity falls short. It also can’t accurately account for the movement of large objects that are close together, such as how the orbit of Mercury is affected by its proximity the Sun.
Albert Einstein’s most consequential breakthrough solved these problems. General relativity holds that gravity is not really an invisible force of mutual attraction, but a distortion. Rather than some kind of mutual tug-of-war, large objects like the Sun and other stars respond relative to each other because the space they are in has been altered. Their mass is so great that they bend the fabric of space and time around themselves.
Read More: 10 Surprising Facts About the 2024 Solar Eclipse
This was a weird concept, and many scientists thought Einstein’s ideas and equations were ridiculous. But others thought it sounded reasonable. Einstein and others knew that if the theory was correct, and the fabric of reality is bending around large objects, then light itself would have to follow that bend. The light of a star in the great distance, for instance, would seem to curve around a large object in front of it, nearer to us—like our Sun. But normally, it’s impossible to study stars behind the Sun to measure this effect. Enter an eclipse.
Einstein’s theory gives an equation for how much the Sun’s gravity would displace the images of background stars. Newton’s theory predicts only half that amount of displacement.
Eddington and Dyson measured the Hyades cluster because it contains many stars; the more stars to distort, the better the comparison. Both teams of scientists encountered strange political and natural obstacles in making the discovery, which are chronicled beautifully in the book No Shadow of a Doubt: The 1919 Eclipse That Confirmed Einstein's Theory of Relativity, by the physicist Daniel Kennefick. But the confirmation of Einstein’s ideas was worth it. Eddington said as much in a letter to his mother: “The one good plate that I measured gave a result agreeing with Einstein,” he wrote, “and I think I have got a little confirmation from a second plate.”
The Eddington-Dyson experiments were hardly the first time scientists used eclipses to make profound new discoveries. The idea dates to the beginnings of human civilization.
Careful records of lunar and solar eclipses are one of the greatest legacies of ancient Babylon. Astronomers—or astrologers, really, but the goal was the same—were able to predict both lunar and solar eclipses with impressive accuracy. They worked out what we now call the Saros Cycle, a repeating period of 18 years, 11 days, and 8 hours in which eclipses appear to repeat. One Saros cycle is equal to 223 synodic months, which is the time it takes the Moon to return to the same phase as seen from Earth. They also figured out, though may not have understood it completely, the geometry that enables eclipses to happen.
The path we trace around the Sun is called the ecliptic. Our planet’s axis is tilted with respect to the ecliptic plane, which is why we have seasons, and why the other celestial bodies seem to cross the same general path in our sky.
As the Moon goes around Earth, it, too, crosses the plane of the ecliptic twice in a year. The ascending node is where the Moon moves into the northern ecliptic. The descending node is where the Moon enters the southern ecliptic. When the Moon crosses a node, a total solar eclipse can happen. Ancient astronomers were aware of these points in the sky, and by the apex of Babylonian civilization, they were very good at predicting when eclipses would occur.
Two and a half millennia later, in 2016, astronomers used these same ancient records to measure the change in the rate at which Earth’s rotation is slowing—which is to say, the amount by which are days are lengthening, over thousands of years.
By the middle of the 19th century, scientific discoveries came at a frenetic pace, and eclipses powered many of them. In October 1868, two astronomers, Pierre Jules César Janssen and Joseph Norman Lockyer, separately measured the colors of sunlight during a total eclipse. Each found evidence of an unknown element, indicating a new discovery: Helium, named for the Greek god of the Sun. In another eclipse in 1869, astronomers found convincing evidence of another new element, which they nicknamed coronium—before learning a few decades later that it was not a new element, but highly ionized iron, indicating that the Sun’s atmosphere is exceptionally, bizarrely hot. This oddity led to the prediction, in the 1950s, of a continual outflow that we now call the solar wind.
And during solar eclipses between 1878 and 1908, astronomers searched in vain for a proposed extra planet within the orbit of Mercury. Provisionally named Vulcan, this planet was thought to exist because Newtonian gravity could not fully describe Mercury’s strange orbit. The matter of the innermost planet’s path was settled, finally, in 1915, when Einstein used general relativity equations to explain it.
Many eclipse expeditions were intended to learn something new, or to prove an idea right—or wrong. But many of these discoveries have major practical effects on us. Understanding the Sun, and why its atmosphere gets so hot, can help us predict solar outbursts that could disrupt the power grid and communications satellites. Understanding gravity, at all scales, allows us to know and to navigate the cosmos.
GPS satellites, for instance, provide accurate measurements down to inches on Earth. Relativity equations account for the effects of the Earth’s gravity and the distances between the satellites and their receivers on the ground. Special relativity holds that the clocks on satellites, which experience weaker gravity, seem to run slower than clocks under the stronger force of gravity on Earth. From the point of view of the satellite, Earth clocks seem to run faster. We can use different satellites in different positions, and different ground stations, to accurately triangulate our positions on Earth down to inches. Without those calculations, GPS satellites would be far less precise.
This year, scientists fanned out across North America and in the skies above it will continue the legacy of eclipse science. Scientists from NASA and several universities and other research institutions will study Earth’s atmosphere; the Sun’s atmosphere; the Sun’s magnetic fields; and the Sun’s atmospheric outbursts, called coronal mass ejections.
When you look up at the Sun and Moon on the eclipse, the Moon’s day — or just observe its shadow darkening the ground beneath the clouds, which seems more likely — think about all the discoveries still yet waiting to happen, just behind the shadow of the Moon.
More Must-Reads from TIME
- How Donald Trump Won
- The Best Inventions of 2024
- Why Sleep Is the Key to Living Longer
- Robert Zemeckis Just Wants to Move You
- How to Break 8 Toxic Communication Habits
- Nicola Coughlan Bet on Herself—And Won
- Why Vinegar Is So Good for You
- Meet TIME's Newest Class of Next Generation Leaders
Contact us at letters@time.com