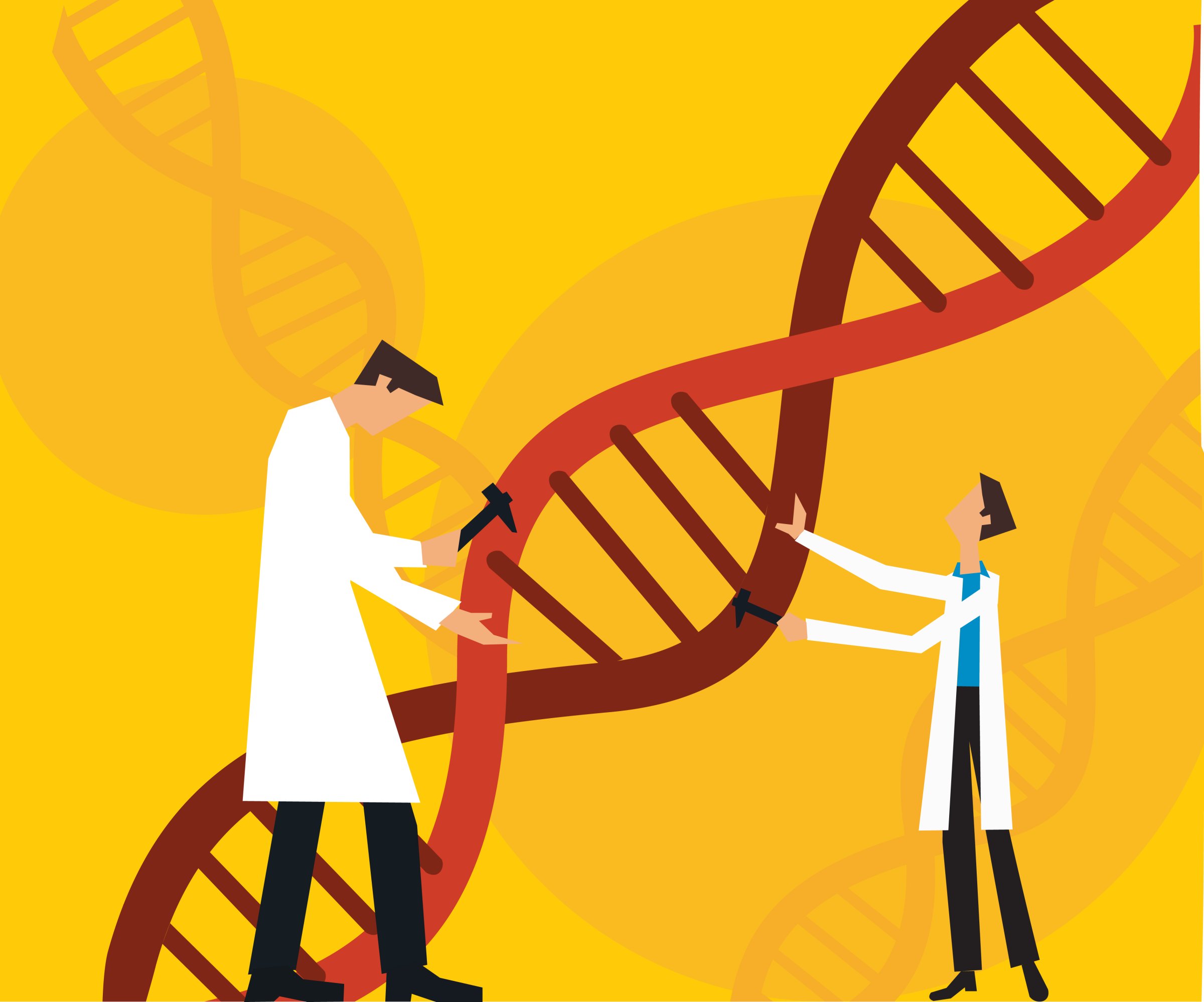
Ever since the human genome was mapped in 2001, scientists have been finding new and novel ways to manipulate it: intervening to remove offending genes or DNA sequences that can contribute to disease, and fixing mutations that can affect people’s health. As remarkable as those advances have been, however, they have only occurred on one dimension—the linear sequence of DNA.
Now scientists report in the Proceedings of the National Academy of Sciences their success in manipulating the genome in 3D. The human genome that’s squeezed into every microscopic cell in the body measures more than two meters long. To stuff it into a space just a few microns wide (the human hair, by comparison, is 40 to 50 microns in diameter) requires some masterful origami-like transformation.
MORE: Don’t Trash These Genes
See the Top 20 Winners of Nikon Small World Microscopic Photography Contest
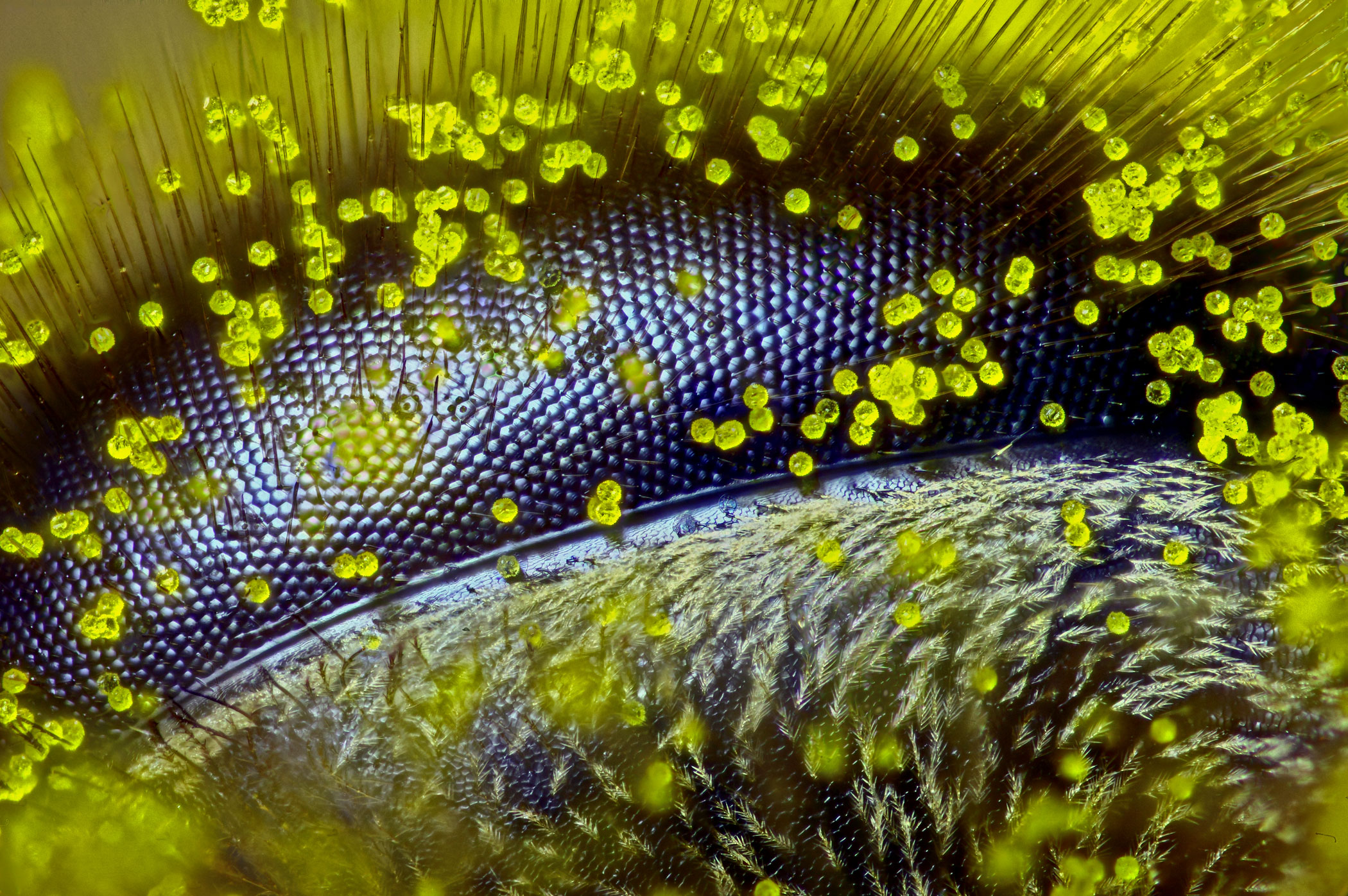

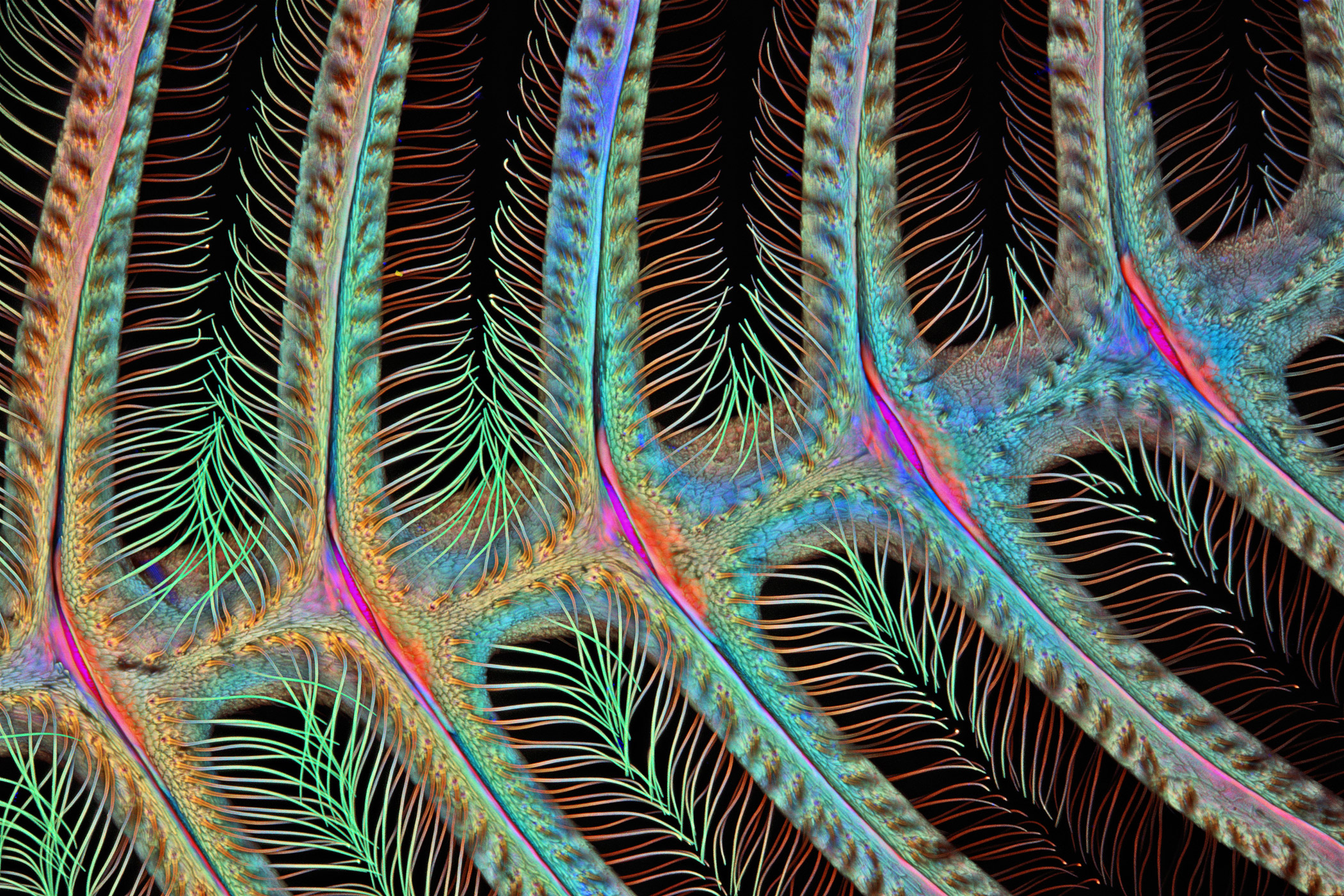

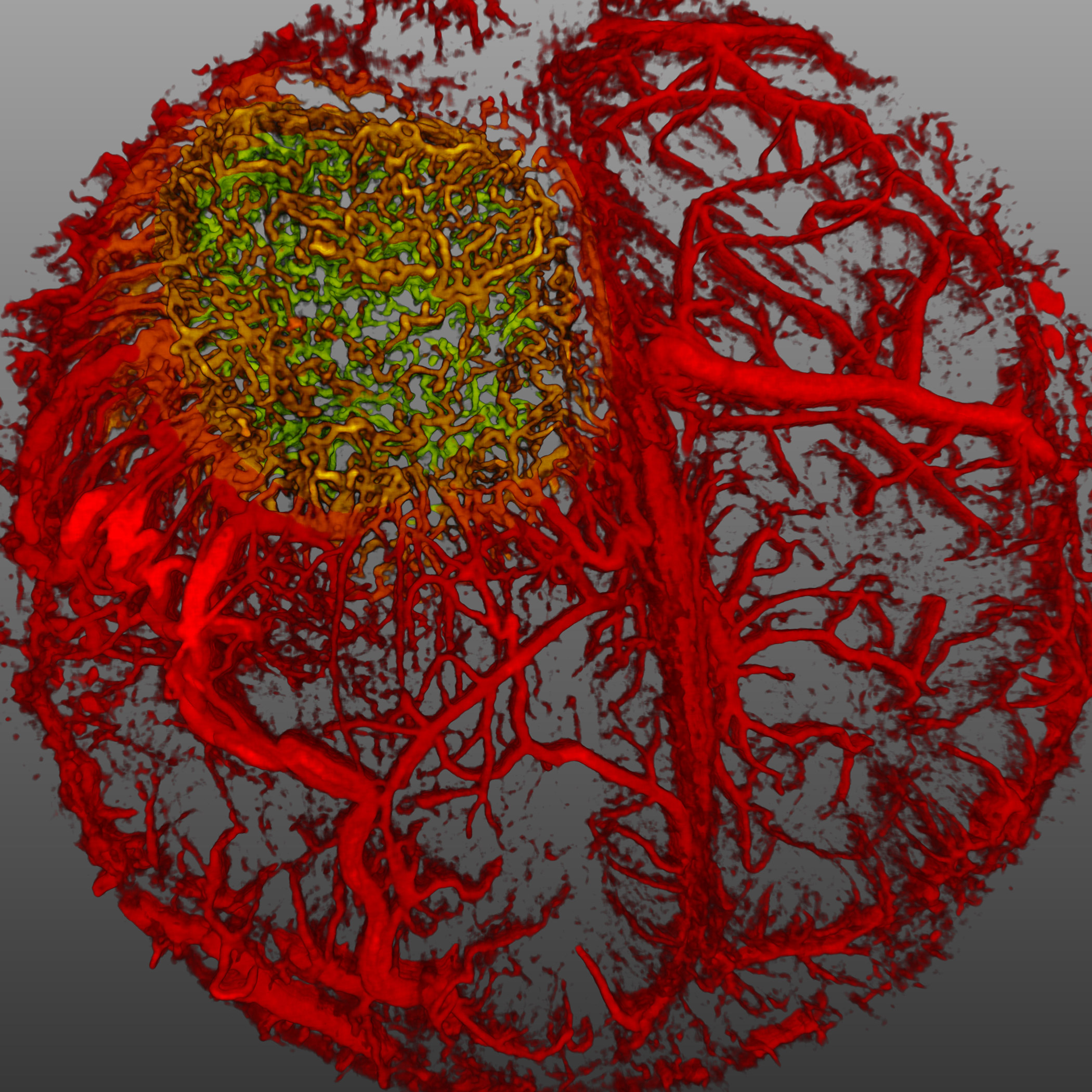

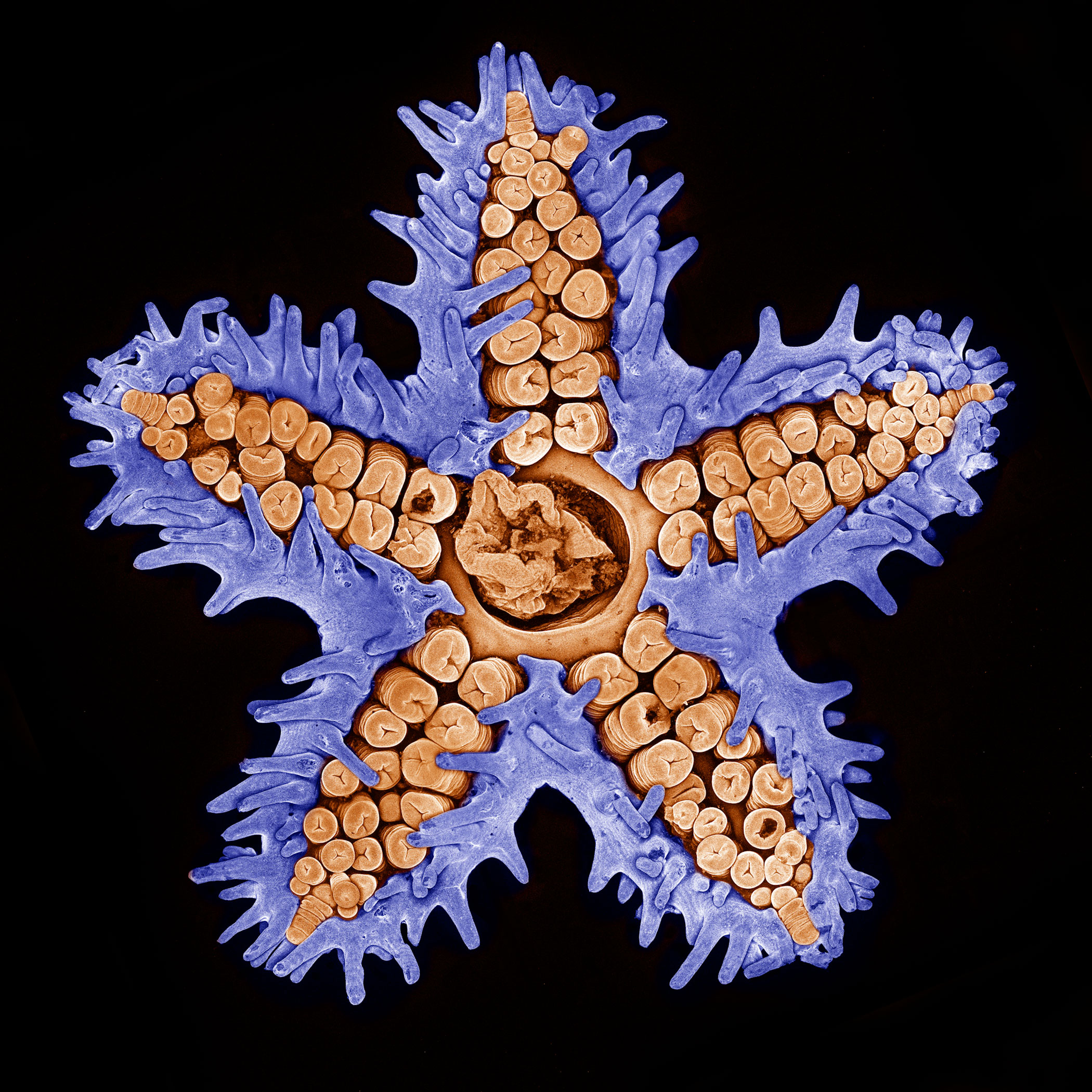
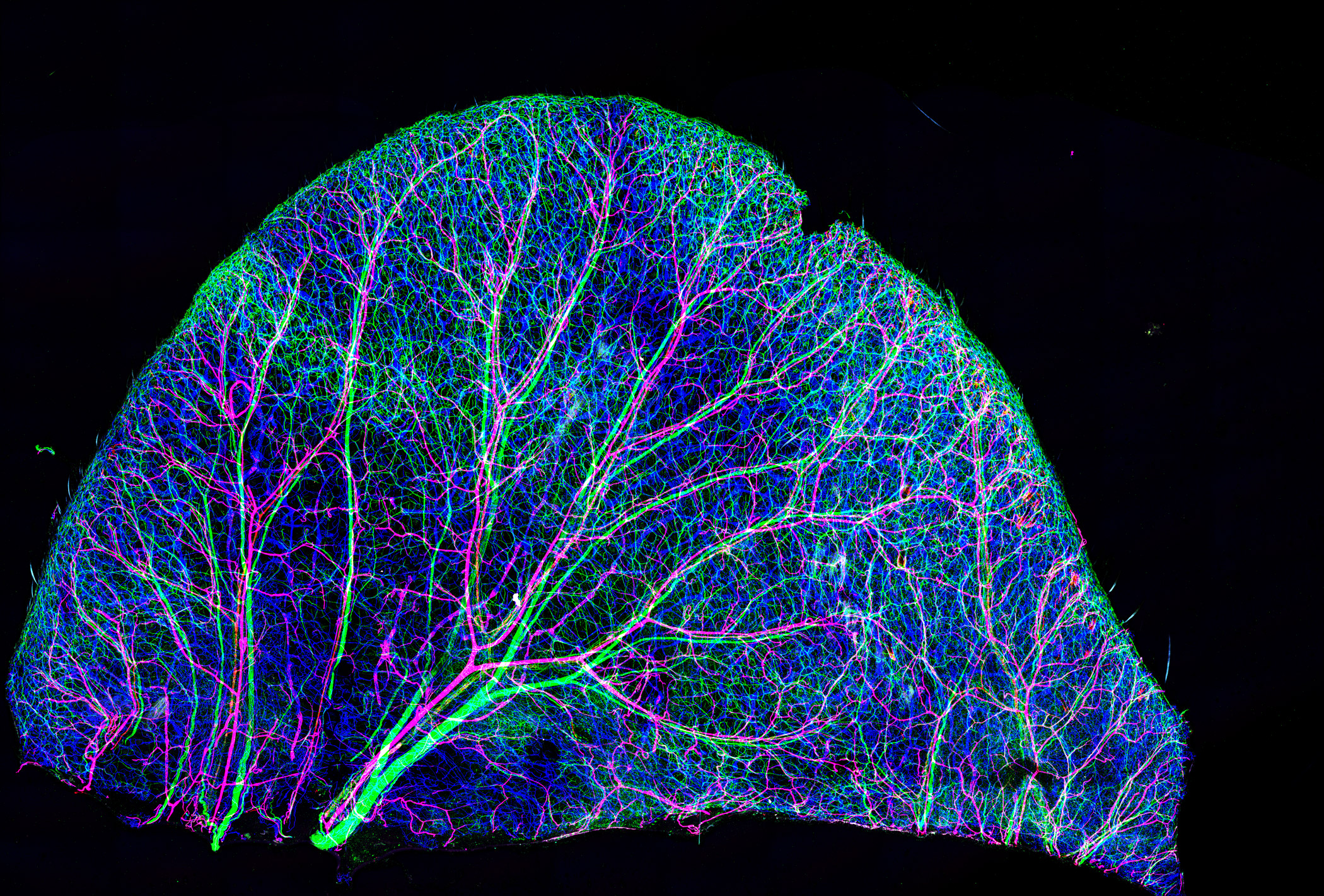


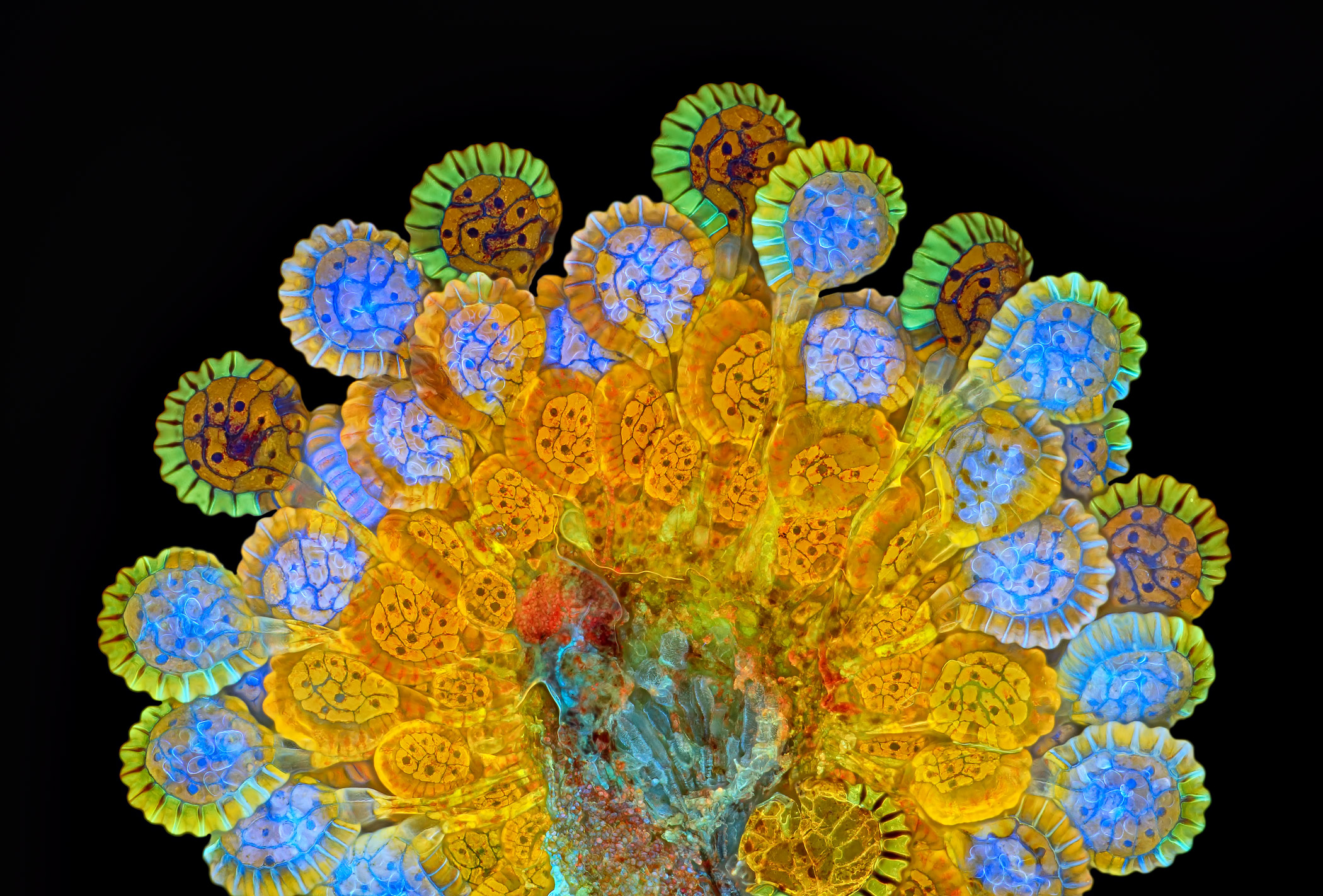


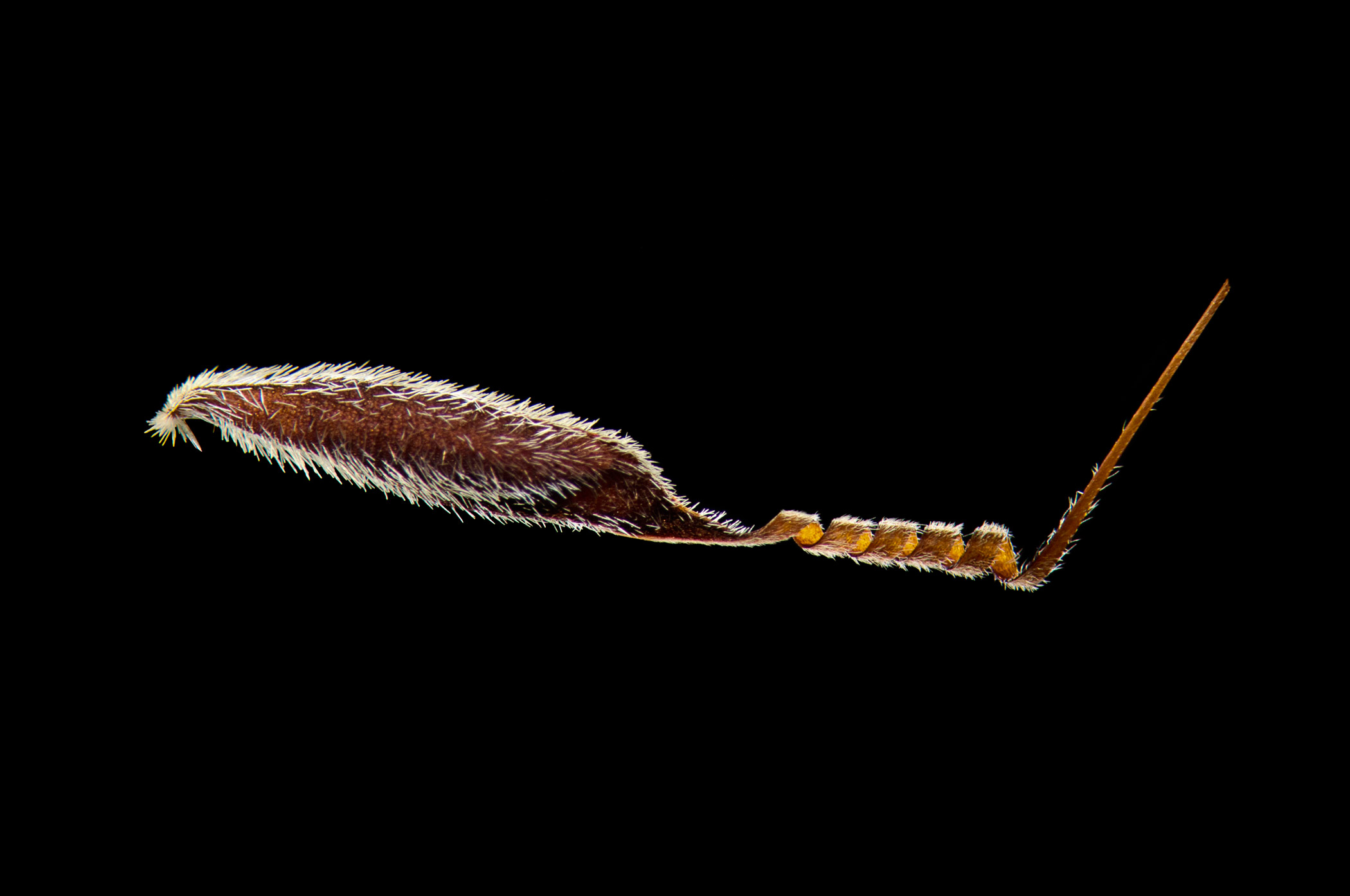
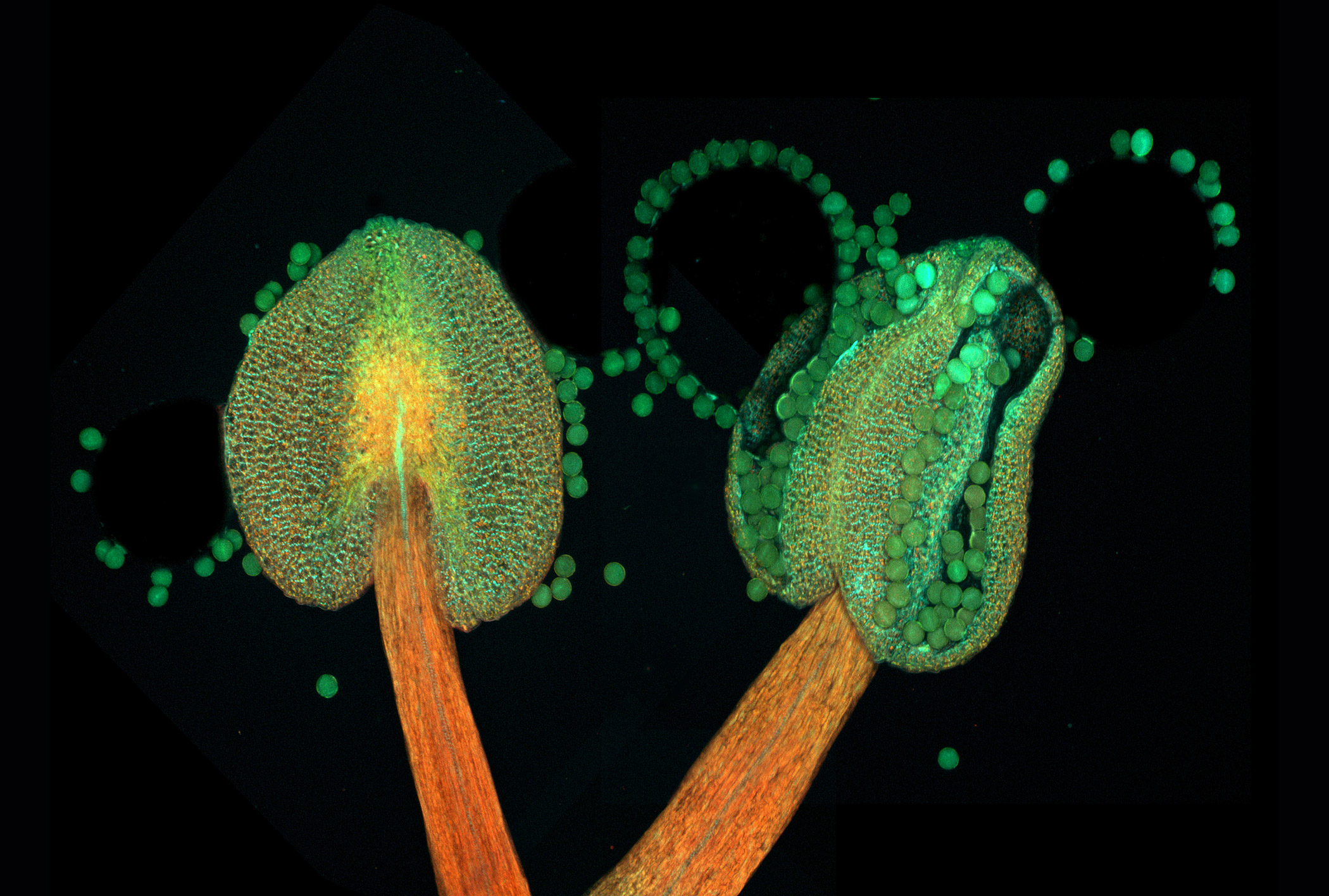
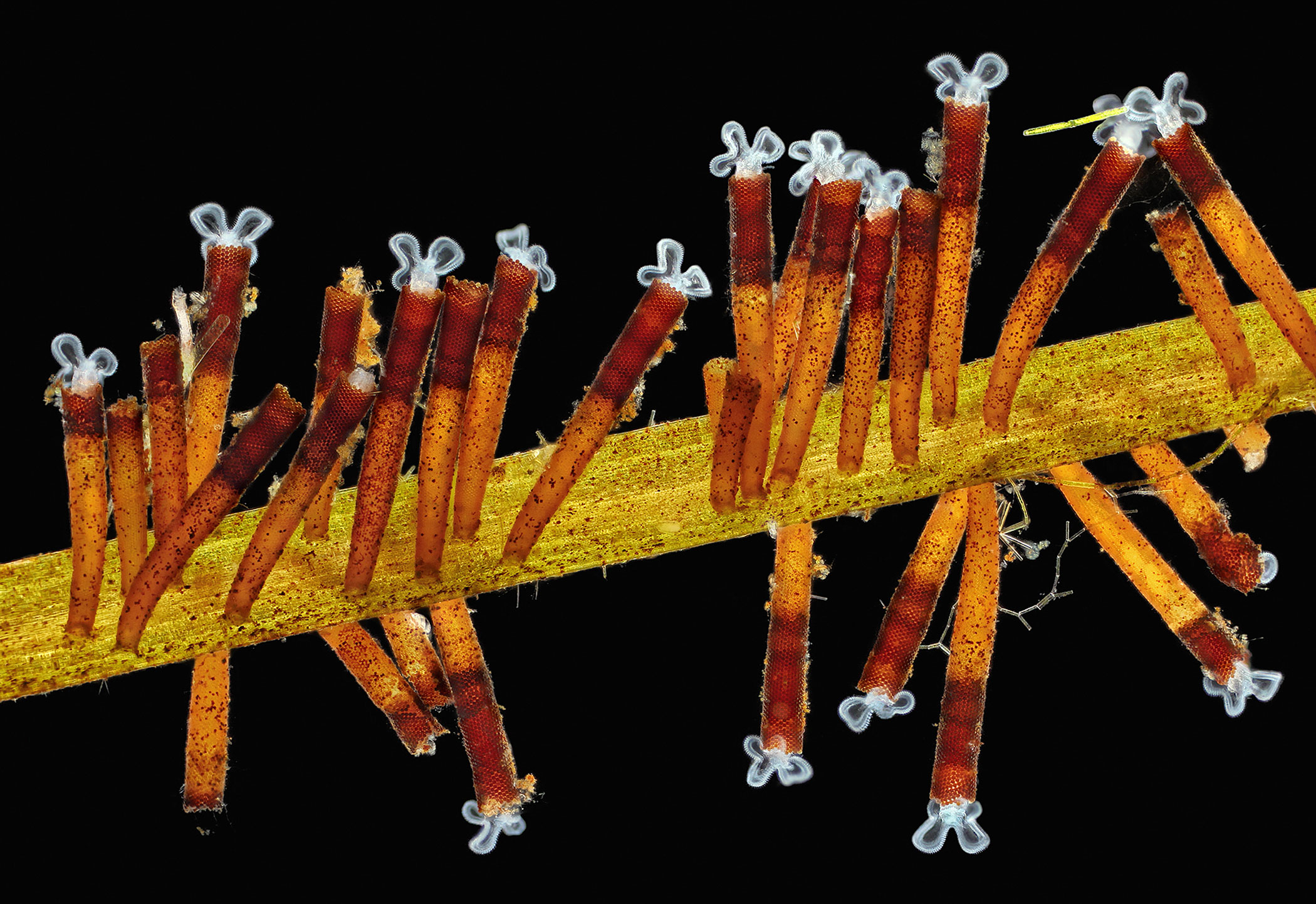
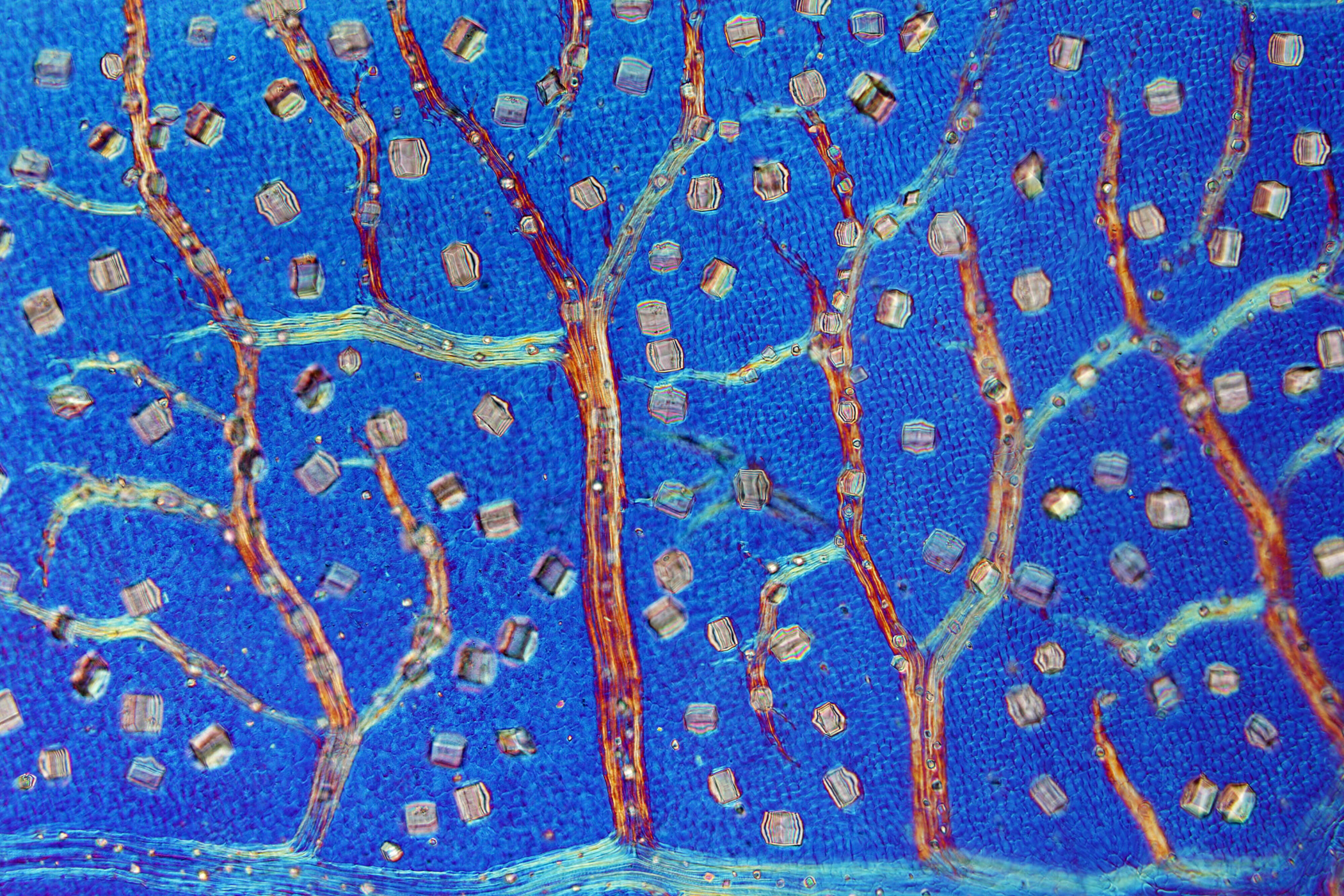


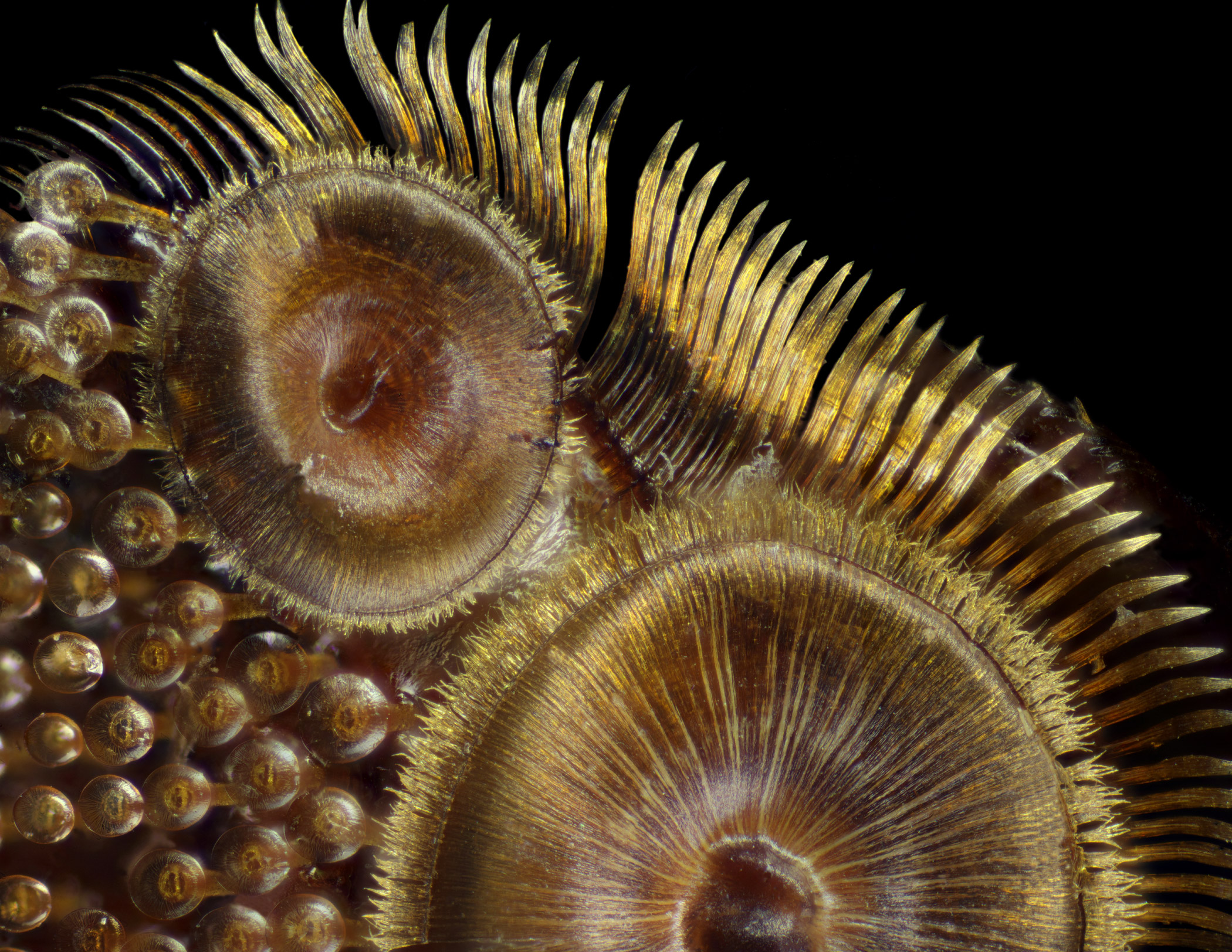
In the study, Erez Lieberman Aiden, director of the center for genome architecture at Baylor College of Medicine and Rice University, and his colleagues describe how DNA performs this shrinking act. It turns out that there is a sequence in the genome—a DNA “word”—that signals when a long string of DNA should turn and form a loop. The end of that loop is signaled by the same word but in reverse, a mirror image of the original. Where these matched-up words appear on the genome determines which genes are exposed in a relatively accessible place and therefore which genes are more active. Loops formed in cells in the heart, for example, will be different from ones generated in skin cells or bone cells.
“We have the same genome in all of our cells, yet cells perform totally different functions,” says Aiden. “That has to do with the fact that different genes are on and off in different cells. How that is managed is in part by the loops of DNA that they form. Think of an origami-like situation—you start with a blank sheet of paper, but whether you can fold that into a hat, plane or crane is a matter of folds. And its function—as a hat, plane or crane—also depends on those folds.”
Not only did Aiden and his colleagues discover the way that loops form, they also conducted experiments to show that they can manipulate where these loops form and potentially change which genes are active and which are silent in specific cells. Conditions like Beckwith-Wiedemann syndrome, for example, may be partly explained by such abnormalities in the way chromosomes loop. Children born with the condition tend to be larger than their peers and develop larger abdomens due to differences in the way that certain genes inherited from both parents on chromosome 11 are expressed.
These findings hint at the potential of treating such conditions, although more work has to be done to better understand how 3D factors affect genetic diseases. But combining the ability to change DNA sequences in both a linear way, as well as a three-dimensional way, could provide a rich new way of treating certain diseases. “By changing the way the DNA is folded, we can change what the genome is doing,” says Aiden. “We can change the function of a cell. In many cases there are multiple ways of getting a condition, and one of them might be 3D mediated.”
More Must-Reads From TIME
- The 100 Most Influential People of 2024
- Coco Gauff Is Playing for Herself Now
- Scenes From Pro-Palestinian Encampments Across U.S. Universities
- 6 Compliments That Land Every Time
- If You're Dating Right Now , You're Brave: Column
- The AI That Could Heal a Divided Internet
- Fallout Is a Brilliant Model for the Future of Video Game Adaptations
- Want Weekly Recs on What to Watch, Read, and More? Sign Up for Worth Your Time
Contact us at letters@time.com